Research
Berezinskii-Kosterlitz-Thouless transition
While true long-range order is prohibited in low-dimensional systems, two-dimensional (2D) systems undergo a phase transition through a vortex-antivortex binding mechanism, known as Berezinskii-Kosterlitz-Thouless (BKT) phase transition. We have recently observed the BKT transition in 2D Bose gases via the interferometric investigation of local phase fluctuations. The observed local fluctuation allows the computation of correlation function and local vortex density, which agree well with the classical-field simulation results performed by theory collaborators [1].
[1] Observation of the BKT Transition in a 2D Bose Gas via Matter-Wave Interferometry. S. Sunami, V. P. Singh, D. Garrick, A. Beregi, A. J. Barker, K. Luksch, E. Bentine, L. Mathey, C. J. Foot. Phys. Rev. Lett. 128, 250402 (2022)

Non-equilibrium dynamics in 2D quantum systems across a critical point
Non-equilibrium physics is an area of great theoretical and experimental interest. Equilibration of classical systems has been described with great success, but there remain open questions about how quantum systems reach equilibrium: what role do local dynamics play in thermalisation, and over what timescale does thermalisation occur? Thermalisation close to a critical point is also interesting since the corresponding equilibrium state is described by universal scaling laws, but it is not known to what extent universality remains valid out of equilibrium. Quantum systems confined to two dimensions (2D) are especially interesting: fluctuations play a large role and prevent true long-range order, but the BKT phase transition to a superfluid at non-zero temperature does exist. We investigate how the non-equilibrium dynamics evolve in the presence of this critical point and compare it with the real-time RG theory predictions [1, 2].
In our apparatus, we can prepare a 2D superfluid in an out-of-equilibrium state via a sudden quench. This is achieved by rapidly splitting the 2D cloud into a double-well potential [3], producing two initially identical clouds which then evolve independently. By later recombining the clouds in time-of-flight we can observe the phase fluctuations and their evolution over time via matter-wave interference (MWI) [3].
[1] Universal Scaling of the Dynamic BKT Transition in Quenched 2D Bose Gases. S. Sunami, V. P. Singh, D. Garrick, A. Beregi, A. J. Barker, K. Luksch, E. Bentine, L. Mathey, C. J. Foot. Science 382, 443 (2023)
[2] Dynamic Kosterlitz-Thourless transition in two-dimensional Bose mixtures of ultracold atoms. L. Mathey, K.J. Günter, J. Dalibard and A. Polkovnikov. Phys. Rev. A 95, 053630 (2017)
[3] Coherent splitting of two-dimensional Bose gases in magnetic potentials. A. J. Barker, S. Sunami, D. Garrick, A. Beregi, K. Luksch, E. Bentine and C. J. Foot. New J. Phys. 22 103040 (2020)

Team
Multiple-RF-dressed double-well potential
RF-dressed adiabatic potentials allow the creation of smooth trap geometry without optical speckles and allows to trap atoms with extremely low heating rate. By applying multiple independently-generated dressing frequencies, we have extended the scope of the RF dressing method and demonstrated the application of three frequencies to create a precisely configurable multiple-RF (MRF) double-well [1].
Our multiple-radiofrequency approach allows for precise control over the double-well potential, including the depth of individual wells and the height of the barrier, and enables the reliable transfer of atoms between the available trapping geometries. Tight confinement along the static magnetic field direction, compared to the other two directions, allows the control of dimensionality with which low-dimensional physics and dimensional crossover can be studied[2]. Utilising differences in gF factors of different atomic species (or internal states), species-selective trapping and manipulation is possible without any change in apparatus (see below).
[1] Ultracold atoms in multiple-radiofrequency dressed adiabatic potentials. T.L. Harte, E. Bentine, K. Luksch, A.J. Barker, D. Trypogeorgos, B. Yuen and C.J. Foot. Phys. Rev. A 97, 013616 (2017)
[2] Coherent splitting of two-dimensional Bose gases in magnetic potentials. A.J. Barker, S. Sunami, D. Garrick, A. Beregi, K. Luksch, E. Bentine and C.J. Foot. arXiv: 2006.06661
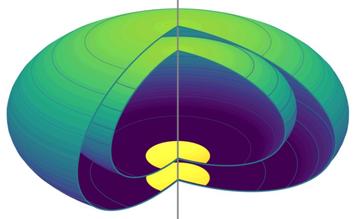
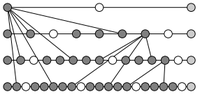
Species-Selective Manipulation of a Multi-Component Quantum Gas
We have realised a scheme in which species-selective control is achieved using magnetic potentials dressed with multiple radiofrequencies (RFs), exploiting the different Lande gF -factors of the constituent atomic species [1,2,3]. The resulting potentials have low heating rates, and are extremely smooth and free from defects. Species with Lande g-factors that differ in sign or magnitude can be manipulated independently. We use a mixture of either two distinct atoms (Rb-87 and Rb-85) or different hyperfine states of the same atom (Rb-87, F = 1 & F = 2).
[1] Species-selective confinement of atoms dressed with multiple radiofrequencies. E. Bentine, T. L. Harte, K. Luksch, A. J. Barker, J. Mur-Petit, B. Yuen, C. J. Foot. J. Phys. B: At. Mol. Opt. Phys. 50 094002 (2017)
[2] Inelastic collisions in radiofrequency-dressed mixtures of ultracold atoms. E. Bentine, A.J. Barker, K. Luksch, S. Sunami, T.L. Harte, B. Yuen, C.J. Foot, D.J. Owens and J.M. Hutson, Phys. Rev. Research 2, 033163 (2020)
[3] Realising a species-selective double well with multiple-radiofrequency-dressed potentials. A.J. Barker, S. Sunami, D. Garrick, A. Beregi, K. Luksch, E. Bentine, C.J. Foot. J. Phys. B: At. Mol. Opt. Phys. 53 155001 (2020)
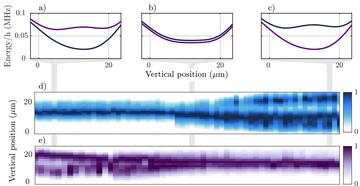
Non-destructive measurement and spin squeezing
In MRF-dressed double-well potential, the dynamics of magnetic spins have different frequency components depending on their trapped location ("left" or "right" well). Utilising this, Voigt detection method yields double-well population information encoded in frequency domain. Theoretical and experimental works are underway to achieve conditional spin squeezing with such a detection method.
Faraday imaging induced squeezing of a double-well Bose-Einstein condensate. E. O. Ilo-Okeke, S. Sunami, C. J. Foot, T. Byrnes. Phys. Rev. A 104, 053324 (2021)
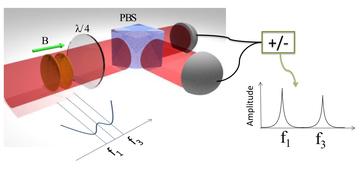
Machine learning optimisation of BEC experiments
To observe the BEC phase transition in dilute gas experiments, extremely low temperatures of tens of nanokelvin are typically required. The techniques used to reach these ultracold temperatures usually include a combination of optical cooling and forced evaporative cooling. Implementing these cooling processes requires the precise sequencing of time-varying magnetic and optical fields using a control computer. The parameter space that describes a typical experimental sequence is large and locating the optimal experimental settings using exhaustive, brute-force searches is unfeasible.
We have applied machine learning methods to the production of quantum gases in our apparatus and have shown they can outperform human optimisation at producing large atom numbers at cold temperatures, even when starting from random initial parameters [1]. Not only do these techniques help us in the lab, but these can also be applied to optimise other aspects of the experimental sequence such as reducing the sequence time, optimising matter-wave interference visibility and preparing cold gases with a specified parameters such as atom number.
[1] Applying machine learning optimization methods to the production of a quantum gas, A.J. Barker, H. Style, K. Luksch, S. Sunami, D. Garrick, F. Hill, C.J. Foot and E. Bentine, Mach. Learn.: Sci. Technol. 1 015007 (2020)
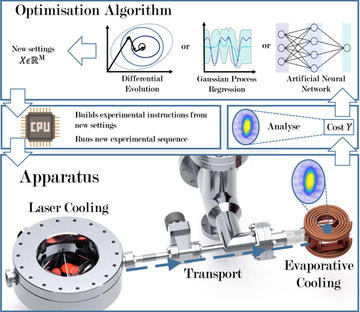
Team
There is a currently a rapid drive towards transferring cutting-edge quantum research into real-life applications, and we are developing practical cold atom sources for these devices. Ultracold atoms have a wide range of applications - including improved atomic clocks, gyroscopes, and sensing of gravitational and magnetic fields - because they are extremely sensitive to external fields and forces.
The majority of ultracold atom experiments use a 2-stage arrangement: atoms are first cooled from room temperature to ~1 millikelvin in a vacuum chamber, then are transported to a neighbouring chamber where they are further cooled and probed. We are engineering the first stage of this process to produce more atoms while reducing its size, using our patent-pending pyramidal arrangement of mirrors (see below). This compact and robust cold atom source can then be integrated into a wide range of practical quantum devices, and the increased atom number will enhance signal-to-noise and repetition rate.
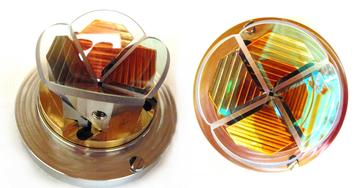
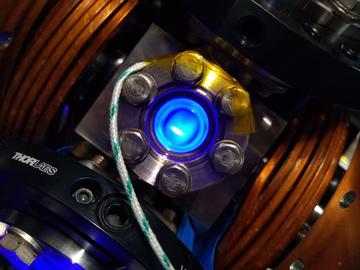